Beyond the Higgs [theoretical particle physics @HIP]
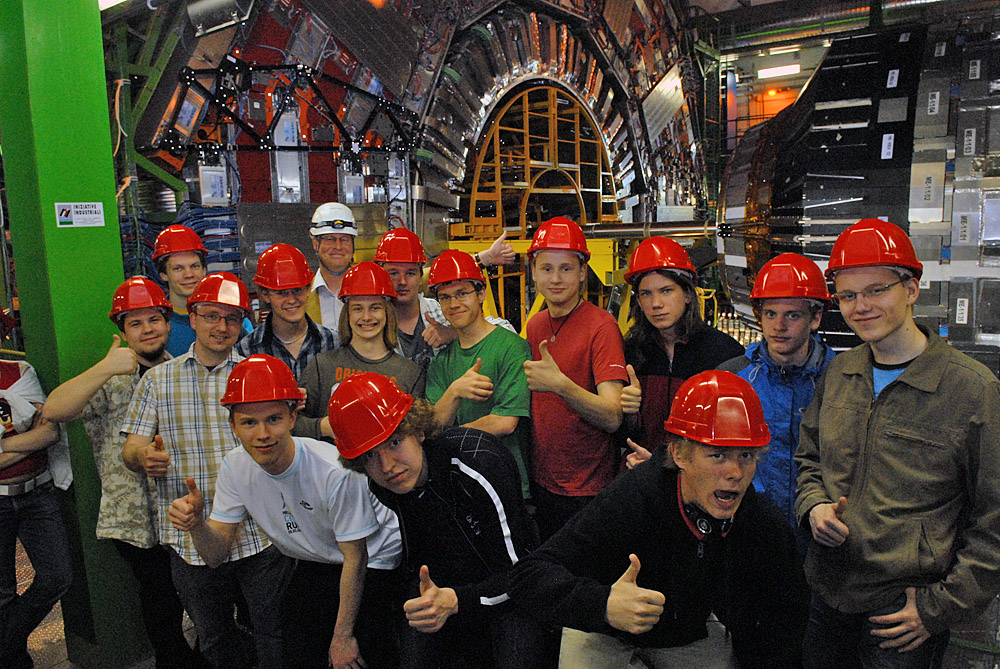
In this blog post, I am going to take you on a short tour of some of the theoretical particle physics work that goes on at HIP. There is so much work going on, across so many different areas, that I can only hope to give you a flavour. My selection of topics is by no means impartial or complete.
Introduction
In July 2012, the two major experiments at CERN, CMS (of which HIP is a member) and ATLAS, announced that they had detected a new particle which had the characteristics expected of the missing, and long-awaited Higgs boson. The Higgs was a missing jigsaw piece of the Standard Model of particle physics, and its discovery finally tied up quite a few loose ends. Most importantly, the “turning on” of the Higgs boson would explain why certain other particles are massive, and why the electromagnetic and weak nuclear forces ceased to be unified a few picoseconds after the Big Bang.
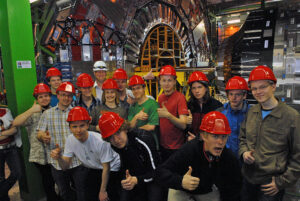
A group of Finnish high school students visiting CMS with Dr. Jaakko Härkönen
Some questions were, however, left unanswered. One nagging question—which happens to interest me in particular—is where all the antimatter in the universe went (if it was ever there at all). Look around you. Look further. In fact, look all the way to the edge of the visible universe. It’s all matter; the antimatter has been destroyed, converted or—just perhaps—it was never there in the first place. And yet, the laws of physics (such as the Standard Model of particle physics) do not really discriminate between matter and antimatter. And what little differences there are don’t do enough to explain where all the antimatter went. So, some new physics is needed.
Electroweak baryogenesis
If we assume that something happened to create the matter-antimatter asymmetry and the universe wasn’t born that way, then something extra has to have happened, early in the universe. The process would have to have been violent, maybe even explosive (so that the antimatter content wouldn’t just naturally creep back as the universe equilibrated again). One way—other equally viable options exist, and are studied at iHIP, too—for this to have happened would be if the Higgs boson turned on through a first-order phase transition, like water condensing from steam. This could have happened extremely rapidly, and the laws of physics outside the expanding Higgs bubbles could have been very different from that inside.
Infant universe blows bubbles from Cosmic Defects on Vimeo.
Unfortunately, the Standard Model of particle physics, as it stands, doesn’t allow this to happen. New physics beyond the Standard Model is needed. This is an area of research in which HIP has a very long pedigree, and remains at the cutting edge. Researchers such as Kimmo Kainulainen in Jyväskylä, and Kari Rummukainen in Helsinki, are world experts in studying these scenarios. Many postdocs, including Oliver Gould and Venus Keus in Helsinki, also work on these models.
An unlikely particle physics experiment: LISA
The additional particles needed to make the above scenario–electroweak baryogenesis–work might be detectable in future particle physics experiments, be they dark matter detectors or new particle accelerators. There is, however, another way that hints of this physics could be seen.
The bubbles of the new Higgs phase that form when the Higgs turns on grow and collide quite explosively, burning up extra energy released as the bubbles expand, and turning it into reaction fronts that surround the bubbles as they grow. These reaction fronts heat the universe—which at that point is a plasma of hot fundamental particles—and set up sound waves that continue to spread even after the bubbles have merged. These large excitations of energy, in turn, create gravitational waves.
Tied with the Higgs boson discovery for physics news of the decade (or even century) was, in my opinion, the first direct detection of gravitational waves announced by the LIGO experiment in February 2016. While that announcement was of gravitational waves from merging black holes, practically any really energetic event in the history of the universe will create gravitational waves.
Furthermore, LIGO’s success gave renewed impetus to other missionsincluding the space mission LISA. This will consist of three satellites in a triangular configuration, with laser beams linking them. As gravitational waves pass through, the distance between the satellites will change slightly, registering a signal.
The size of the Higgs bubbles, and therefore the wavelength of those sound waves, happens to be the perfect size to be seen by the LISA mission. Indeed, the science case for LISA discusses exactly this sort of scenario as one of the signals they will be looking for, alongside signals from white dwarf binaries in the Milky Way and from supermassive black holes.
At HIP we are working to make accurate predictions of what LISA will see for a wide range of scenarios. Several HIP physicists are full members of the LISA collaboration, including Mark Hindmarsh, Kari Rummukainen and David Weir. Furthermore, we hosted a workshop of the LISA Cosmology Working Group in June of 2017, bringing dozens of physicists from across Europe and beyond to discuss the electroweak phase transition and other processes in the early universe that might be seen by LISA!
Ending
This has necessarily been a highly selective list of some physics that I work on with my colleagues at HIP, and which involves researchers in both Jyväskylä and Helsinki. Being part of an institute which combines particle physics experiments with cutting-edge research in theoretical physics makes for a stimulating and exciting workplace in which to study the early universe.
David Weir
2 Responses
[…] yliopistolla kollegani Mark Hindmarsh ja David Weir ovat kunnostautuneet näiden törmäysten tarkassa tutkimisessa. He ovat ensimmäisinä maailmassa laskeneet, millaisia […]
[…] gravitational waves from violent events very shortly after the Big Bang, as David Weir explained in his blog post here last […]