Beyond the Standard Model? Neutrinos!
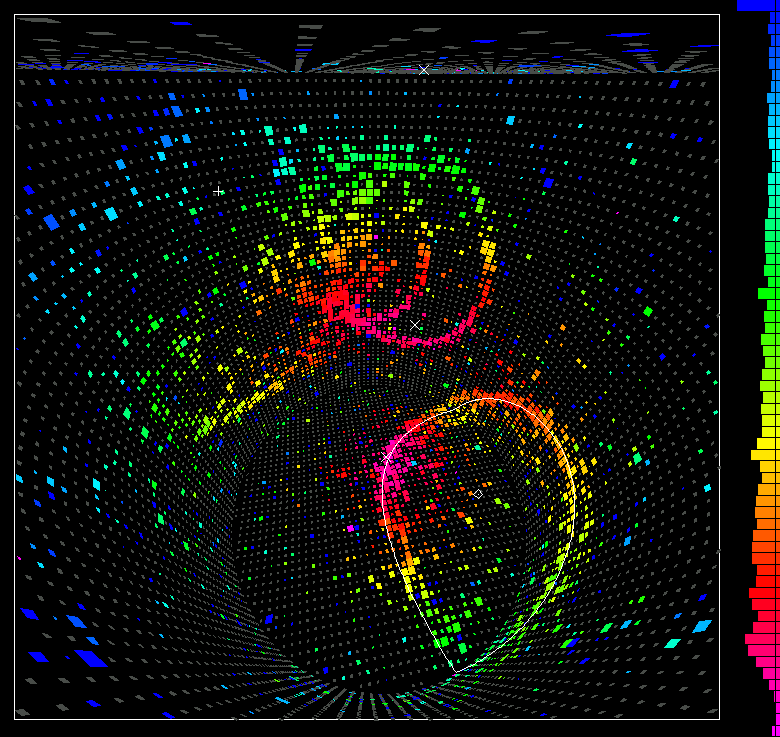
Neutrinos were ”invented” by Pauli in 1930 as a ”desperate remedy” to explain the beta-decay spectrum without giving up energy conservation. That was a time when inventing particles was almost sinful, and Pauli himself called the proposal “incredible”. Perhaps he himself did not fully believe in it, since he wrote it in a letter, not a scientific paper. Or maybe he was just testing the reaction of the fellow physicists to such an unconventional remedy. The letter was addressed to the radioactive group at a regional meeting in Tübingen, in which Pauli could not participate on account of a ball in Zurich…
Very soon, neutrinos became full-fledged members of the realm of fundamental particles, especially after Fermi included them in the first theory of beta decay in 1934. This theoretical promotion did not make them less elusive – during the same year, Bethe and Peierls showed that the estimated cross-section of neutrinos was so small, that they were practically undetectable. Nevertheless, this did not deter the experimentalists who, in desperation – again! – were ready to use as source of neutrinos a hydrogen bomb similar to the one detonated at the end of the Second World War. Luckily for the history of particle physics, this extreme and ethically doubtful source was not necessary: neutrinos were discovered in an experiment led by Cowan and Reines, in 1956, using a reactor source.
Neutrinos have shown up in many revolutionary ideas and experiments which led to the formulation of the Standard Model and went beyond it. Quite a number of Nobel Prizes in Physics are dedicated to neutrino research: in 1988 to Lederman, Schwartz and Steinberger for the discovery of muon neutrino, in 1995 to Reines for the discovery of electron neutrino, in 2002 to Davis and Koshiba for the discovery of cosmic neutrinos, 2015 to Kajita and McDonald for the experimental discovery of neutrino oscillations and conversion in matter. The discovery of neutrino oscillations is the most remarkable achievement of physics beyond the Standard Model. This phenomenon signals the fact that neutrinos are massive and they mix, in contrast with the Standard Model massless neutrinos. Massive neutrinos bring about other puzzling questions, regarding their nature as Dirac or Majorana particles, a dilemma that is hoped to be solved by neutrinoless double beta decay experiments. Plausible mechanisms of leptogenesis indicate the heavy neutrinos, of either Majorana or Dirac type, as responsible for the baryonic asymmetry of the Universe. Neutrinos play a crucial role in modern cosmology and the PTOLEMY experiment is planned for confirming the cosmic neutrino background. The neutrinos are part of the presently much discussed hypothesis of Lorentz invariance violation based on the observation of gamma-ray bursts along with neutrino emission, with the neutrino reaching the detectors before the photons. Though certainly Lorentz invariance violation looks too extreme a hypothesis when so much is not known about the sources of the bursts and about what can happen during the propagation. There are more things in heaven and Earth, Horatio, / Than are dreamt of in your philosophy.
In 1957, Bruno Pontecorvo put forward the idea that neutrinos oscillate into antineutrinos, in the fashion of neutral kaon mixing previously introduced by Gell-Mann, Pais and Piccioni in 1955. This was the time when the Standard Model was far from being established, such that massive neutrinos were not regarded as a transgression. The same year, actually, Landau, Lee and Yang, and Salam proposed the theory of the massless two component neutrino. Interestingly, the two contradictory hypotheses survived and flourished along each other until the end of the 20th century. Pontecorvo kept on refining the idea of oscillations. In 1968, together with Gribov, he proposed that the flavour oscillation between electron neutrino and muon neutrino, which had been discovered in 1962, was the solution for the solar neutrino problem. The paper of Gribov and Pontecorvo fixed the paradigm of neutrino oscillations in vacuum in a form that has survived to this day. (Actually, the application they suggested was latter understood to be not quite so. Instead, solar neutrinos conversion takes place mainly by an adiabatic process in matter, as described later by Mikheyev, Smirnov and Wolfenstein). In 1975, Pontecorvo was joined by Bilenky in a life-long collaboration, which started with a quark-lepton analogy for neutrino oscillations and continuously shaped the field of neutrino oscillations in vacuum over many years.
In spite of the concentrated effort and several ingenious theoretical solutions, there are paradoxical features and questions on whose answer there is still no consensus, such as: how are the flavour neutrino states supposed to be defined? are the flavour states momentum eigenstates? is it necessary that the massive neutrinos which mix have equal energies? are the flavour states physical and in which sense? These are fundamental issues arising about any oscillation process.
I learned about these things directly from Samoil Bilenky, who taught a course on neutrino physics during his visit to Helsinki in the spring of 2000. By that time, the Super-Kamiokande results on vacuum oscillations of atmospheric neutrinos were known and the results of the Sudbury Neutrino Observatory (SNO) experiment on solar neutrino conversion were to come in two years. It was a time of glory and excitement for neutrino physics…
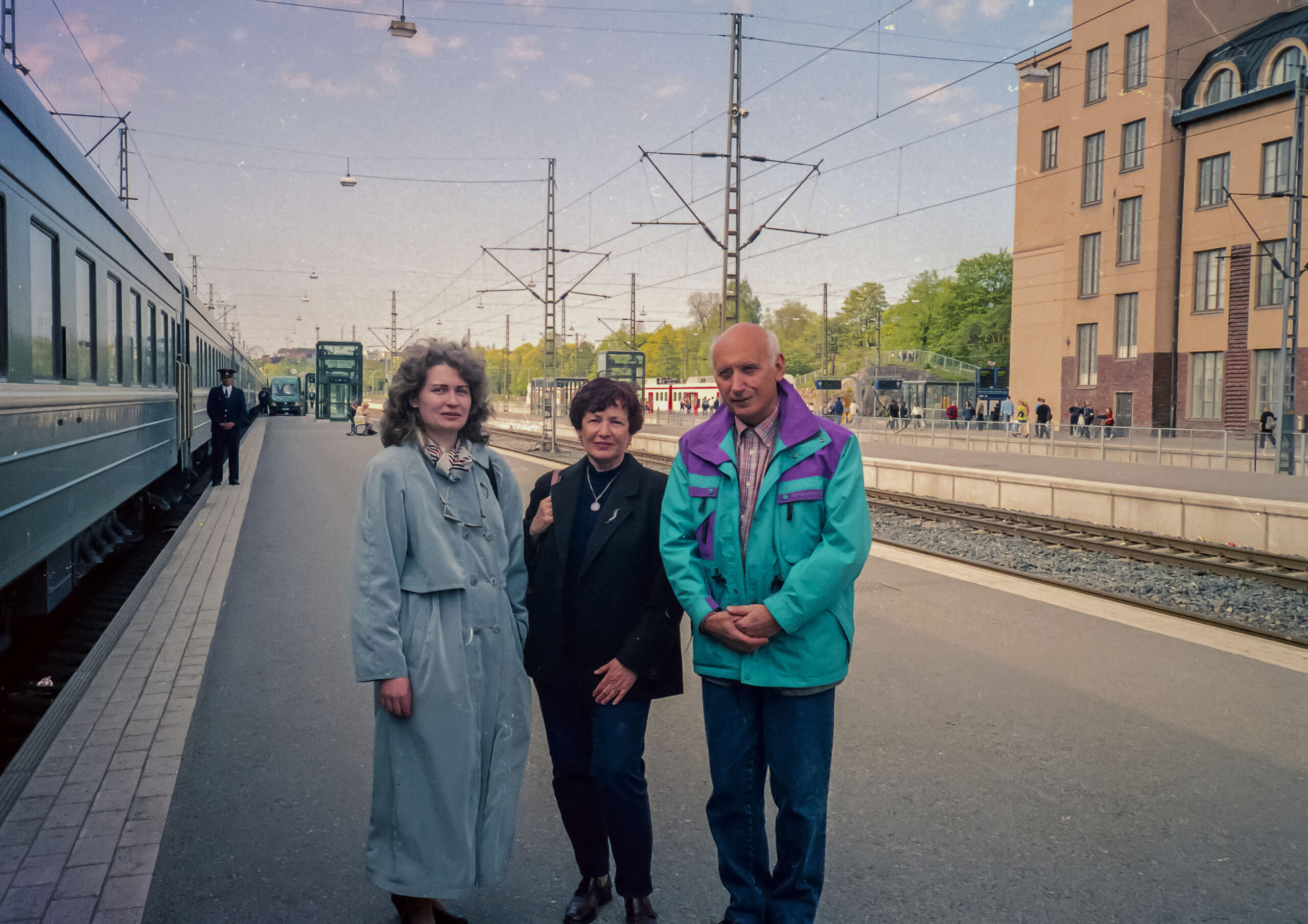
I befriended Samoil and his wife, Sofia, during the summer of 2000. Nevertheless, science-wise, the most pressing questions for neutrino physicists at that moment were of a phenomenological nature and I preferred more mathematical research. My interest in neutrinos was raised again by Samoil in 2012, when I visited him at Dubna. We got to discuss afterwards by email various neutrino problems and his views were always sharp and illuminating. After the 5th of November 2020, I have often missed our correspondence… Back to 2012, Samoil was then absorbed in the analysis of some hypothetical precision tests for neutrinos and explained that the nature of the flavour neutrino states vs. mass eigenstates was not clearly understood. Now, this relation is truly the cornerstone of the oscillation process and it looked to me quite amazing that a central issue like that was still subject for debate. Moreover, it was a wonderful quantum field theoretical problem!
I started to think about it, unsuccessfully at first. But never quite dropped it. Several years later, I got involved more seriously in research on particle oscillations through another route, namely the hypothetical neutron-antineutron oscillations. Studying neutrons, I got closer to neutrinos. And then I understood that the key problem of neutrino oscillation was the coherence of the massive neutrino superpositions that supposedly represent the flavour neutrinos. If the superposition is incoherent, there are no oscillations. The standard theory says, like a mantra, that “flavour neutrinos are emitted and detected as coherent superpositions of massive neutrino states with different masses”. This is a good example of a statement that becomes “true” by repetition – practically all the books and articles repeat it, though it has never been proven! No wonder, because it cannot be proven. On the contrary, quantum field theory is quite adamant that particle states of different masses can never be emitted or absorbed coherently. Do we need a novel, unconventional quantum field theory, or does this mean that neutrinos do not oscillate in vacuum? The standard explanation of this implausible coherence is that massive neutrinos are emitted as wave packets, and as long as the wave packets have some spatial overlap, there is coherence. This is a slippery slope towards more trouble with fundamental theory, and anyway it does not solve the problem: the wave packets have internal coherence, being “coherent states” in the sense of quantum mechanics, but there is still no way to prove that wave packets corresponding to particles of different masses have any coherence between themselves.
The word “coherence” with its derivates has already appeared too many times above. This is because it shows up a lot in physics, with various meanings depending on the context. In 1978, Wolfenstein described the neutrino oscillations in matter making use of the coherent scattering of electron neutrinos with electrons. Matter acts as a refractive medium for the neutrinos, changing their potential energy and modifying the oscillation pattern so much, that in certain conditions the conversion can become resonant. So why not treat the vacuum as a medium, in which massless neutrinos suffer coherent scatterings, which modify not only their potential, but also their flavour? Any mass term in modern particle physics reflects an interaction – for Dirac neutrinos, for example, it is the interaction with the constant scalar background field represented by the vacuum value of the Brout-Englert-Higgs field. In this picture, the coherence emerges naturally like in a quantum mechanical two-level system, in which the interaction between the energy states keeps the system in oscillation. It turns out that the neutrino oscillation is quite clearly a quantum field theoretical process of coherent scattering, treated formally with the tools of quantum mechanics. Conceptually, this is more satisfying than two or three particle states propagating freely and chasing each other until they lose sight of one another. This novel mechanism of neutrino oscillations was published this year in Phys. Lett. B.
This description of massive and mixed neutrinos offers a consistent framework for studying, besides oscillations, also flavour violation in the interactions of neutrino – a phenomenon usually ignored, but which may influence the analysis of oscillation experiments and the extraction of the mass and mixing parameters. Moreover, understanding coherence is equivalent to understanding decoherence, and the latter is a key element for oscillations, for the evaluation of the cosmic neutrino background based on cosmology constraints, and for decoding the neutrino signals from supernova explosions. We are studying these aspects at the moment together with Markku Oksanen and Nico Stirling within our HIP theory project. After 20 years, in 2020, I had the opportunity to introduce a full course on neutrino physics in the curriculum of the Master’s Programme in Particle Physics and Astrophysical Sciences at Helsinki – we trust that our research will attract more young people entranced by physics beyond the Standard Model, by particle oscillations and by the elusive neutrinos.
Anca Tureanu
University Lecturer, Project Leader @ HIP (HEP BSM)
Department of Physics, Helsinki Institute of Physics
University of Helsinki