Materials for future particle colliders
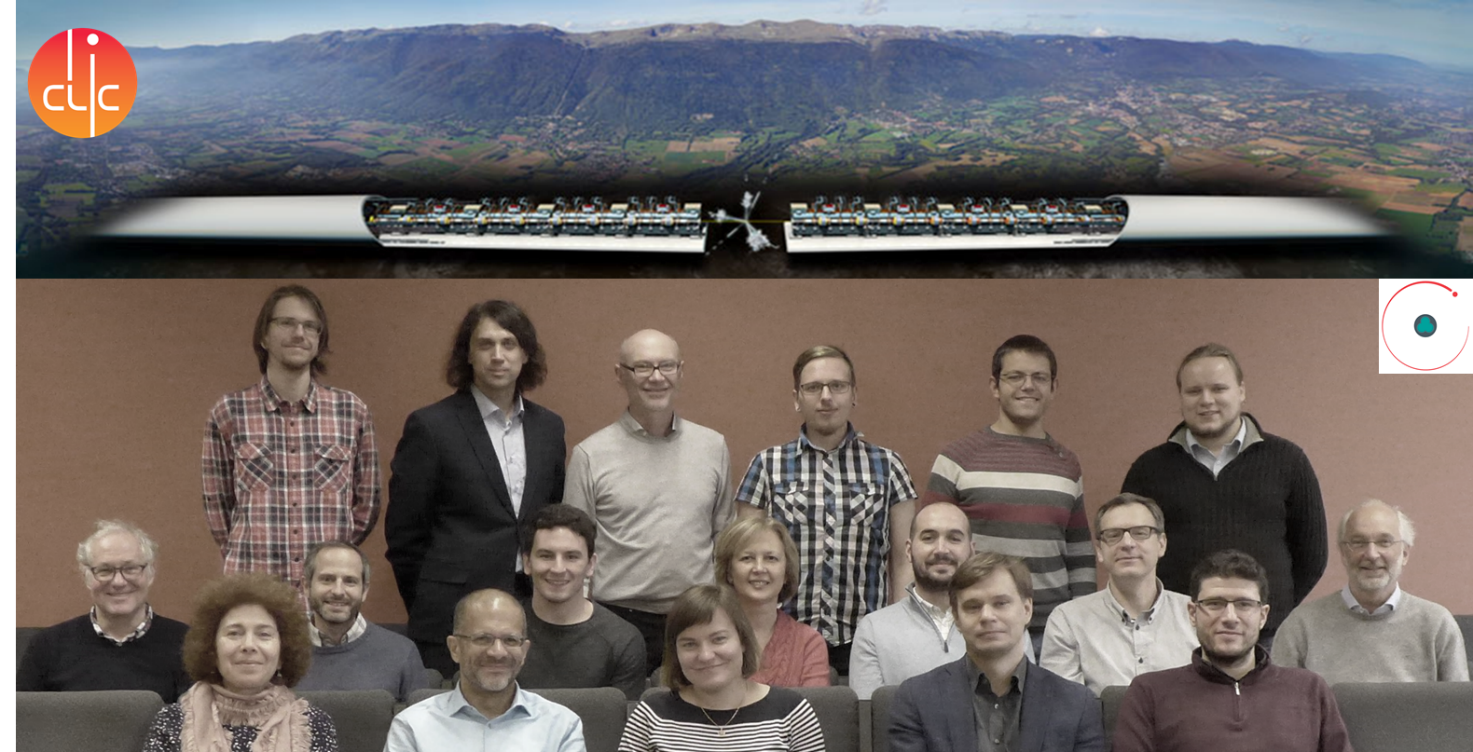
Discovery of the Higgs particles in 2012 became possible because of the tremendous international collaborative effort of a large group of high-energy particle and accelerator physicists and engineers, who enabled the construction of the unprecedentedly powerful 13 TeV Large Hadron Collider, LHC. Yet, not all the mysteries of the Universe were unrevealed by this unique machine. What is the nature of the dark matter? Why does matter prevail over antimatter? What are the neutrino masses? Answering all these questions requires pushing the energy frontiers even further, beyond the current limits. Scientists are looking for alternative solutions, proposing new design studies covering different energy ranges for new discoveries.
One of the design studies at CERN for more precise and accurate study of Higgs particles is the new Compact Linear Collider. The concept of CLIC is a TeV-scale high-luminosity linear electron-positron collider that is aimed to be built in stages, at center-of-mass energies 380 GeV, 1.5 TeV and 3 TeV. CLIC uses a novel two-beam acceleration scheme, in which 12 GHz accelerating structures are powered via a high-current drive beam. The development of this technology benefits many other fields based on use of particle accelerators. Among these are medical accelerators for cancer treatment and free electron lasers. The efforts of many international experts in different fields, including the group of Materials scientists at HIP, have resulted in a thorough optimization of CLIC accelerators, increasing energy efficiency for the 380 GeV stage and significant cost reduction that is estimated at the level of 6 billion CHF. A wide range of CLIC physics studies demonstrated the full CLIC physics potential. It was shown that each of the three energy stages build up the knowledge step-wise. It will be possible to measure Higgs width and couplings, top-quark properties, Higgs self-coupling, direct searches etc. The projected start of the tunnel construction in 2026 may enable the first beams by 2035.
The European Strategy for Particle Physics of 2013 (CERN Council, European Strategy Session of Council, 30 May 2013, CERN-Council-S/106 (2013)) states: “CERN should undertake design studies for accelerator projects in a global context, with emphasis on proton-proton and electron-positron high-energy frontier machines. These design studies should be coupled to a vigorous accelerator R&D programme, including high-field magnets and high-gradient accelerating structures, in collaboration with national institutes, laboratories and universities worldwide.” In response to this, a Future Circular Collider (FCC) study was launched at CERN. This project aims to build a large collider of 80 to 100 km in circumference (for comparison, the circumference of LHC is “only” 27 km).
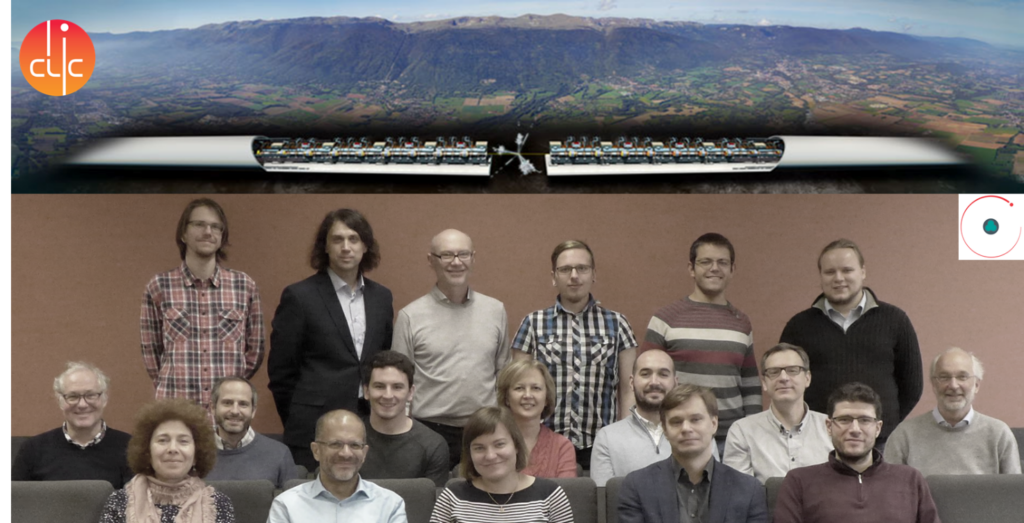
International team, working together to decipher the mechanisms of vacuum arcing, includes the groups from CERN, HIP, Hebrew University of Jerusalem and University of Uppsala. In the background, the artistic vision of CLIC built under the Jura Mountains near CERN.
The physics opportunities within FCC include electron-positron (FCC-ee), proton-proton (FCC-hh), electron-proton (FCC-eh) and heavy ion programmes. It will cover the studies of electroweak, Higgs and strong interactions, the top quark and flavor as well as search for phenomena beyond the Standard Model. The same infrastructure (including building of an expensive tunnel) can be used for different FCC programmes. The first programme, FCC-ee is a high precision instrument to study the Z, W, Higgs and top particles, operating at multiple center-of-mass energies, from 91 GeV to 365 GeV. The FCC-hh will reach 100 TeV center-of-mass energy for proton-proton collision and 39 TeV for Pb-Pb collision. Optionally, the FCC-eh will generate the 50 TeV proton beam to collide with the 60 GeV electrons.
All this impressive physics is not possible without the expertise of the people from many different fields involved in the building of the ambitious machines. Rather unexpectedly, materials physics happened to be in the core of the cost-efficiency of the construction. One of the largest part of the construction costs is the accelerating structures, which are electromagnetic waveguides made of industrial Cu. However, these are needed in a quantity that can match the length of a multi-km accelerator! The accelerating structures of the μm precision geometry are designed to face unprecedented high-gradient electromagnetic waves to carry bunches of GeV or even TeV accelerated particles. It is important to minimize the failure probability of these structures, while push the value of the fields as high as possible. One of the most mysterious phenomena known as vacuum arcing sets physical limits on how high electric fields at a metal surface can be raised. Understanding the cause of this phenomenon can push the working limits higher up, improving the performance of the acceleration, while reducing the costs and possible failure routes. The theoretical model incorporating multiphysics processes on multi- length and time scales, which we develop at HIP, aims to decipher the complex phenomenon, helping to set up a control over the detrimental and wasteful power consumption source.
Long-established expertise in the physics of radiation effects at the Accelerator Laboratory of the University of Helsinki places the group also in the right position to deal with the problems of high flux proton and heavy ion irradiation on materials of beam facing components for future FCC accelerators. On the other hand, huge superconducting magnets build all around the FCC circumference require a very thorough analysis of the quality of the superconducting films. Here again, the Helsinki Accelerator Laboratory is able to offer the unique opportunity of positron annihilation measurements combined with the theoretical modelling of the thin film growth. Joining efforts with the accelerator physicists and engineers, the materials scientists can help to expand the frontiers of high-energy physics beyond the imagined!
Flyura Djurabekova
Prof. in Materials in Extreme Environments
Department of Physics and HIP, University of Helsinki