The discovery of the Odderon
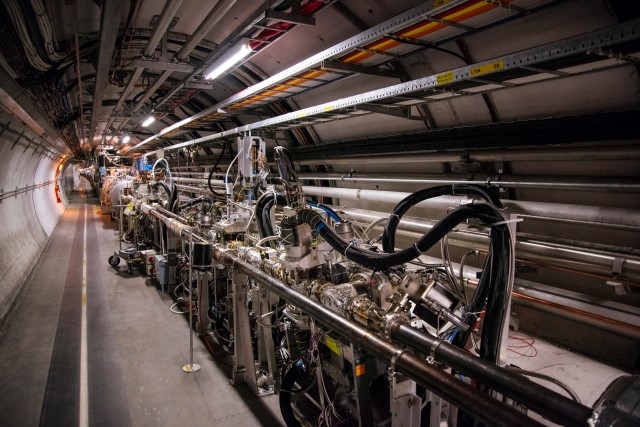
Elementary particle physics is about understanding the smallest constituents of matter and their interactions. To do so larger and larger particle colliders are being built to probe the structure of matter at smaller and smaller distances. Currently, the largest particle collider is CERN’s (European Particle Physics Laboratory) Large Hadron Collider (LHC) that collides protons at an impressing collision energy of 13 Tera electron volt (TeV), corresponding to the energy that an electron would obtain if exposed to an electric field of 13 trillion volts! In 2012, the Higgs boson, the last missing piece to complete the Standard Model, the theory of the elementary particles and their interactions, was discovered at the LHC. But we know that there are many phenomena that the Standard Model cannot provide an answer to.
For that reason physicists continue to search for signs of new, yet unknown, physics or particles in the high energy collisions of the LHC that could potential provide answers to these unanswered questions. But the LHC can also be used to discover much lower energy i.e. larger distance phenomena like “exotic” particles or compounds that can be accommodated in the Standard Model. An example is the discovery of the Odderon, which strongly relied on the measurements of elastic proton-proton collisions by the TOTEM experiment at the LHC. What is an elastic collision? An elastic collision is a collision, where the two protons softly bounces of each other and continue onwards only with a slight change of their direction. TOTEM measures the elastic protons very far away (210-220 meters) from the collision point at a very close distance (a few millimetres) from the outgoing proton beam using special movable devices called Roman Pots, seen in Figure 1.
What is the Odderon?
The Odderon consists of three gluons. Gluons “glue” the quarks together to “hadrons”, particles that are held together by the strong interaction, like the proton and the neutron. Gluons are the mediators of the strong interaction between quarks in a similar way as the photon, the particle which light is made of, is the mediator of the electromagnetic interaction between electrically charged particles. There is however a significant difference: contrary to the photon, the gluons can interact with each other. The gluons themselves carry the charge of the interaction, “colour”, they are mediating. Colour comes in three variants: usually dubbed “green”, “red” and “blue”. Having colour makes quarks and gluons special, they cannot exist by themselves as free particles. They can only exist in colour-neutral combinations made up of all the three colours or by colour-anticolour pairs.
All this is described by the quantum theory of strong interaction, Quantum Chromodynamics (QCD) that together with the quantum theories of electromagnetic and weak interaction make up the Standard Model. Currently, we know that QCD is an accurate description of the strong interaction of quarks and gluons by a multitude of measurements at different colliders. QCD also predicts that there should exist particles made up only of gluons, “glueballs”. So far no conclusive evidence of glueballs has been found despite large efforts over the last 50 years.
QCD also predicts that colourless compounds made up only of gluons should be exchanged during the interaction of particles. In the pre-QCD theory of strong interaction, these compounds were called “Pomeron” and “Odderon”. They differ in the sense that the Pomeron interacts identically with particles and antiparticles, whereas the Odderon interacts in opposite ways with particles and antiparticles. The Odderon was predicted in 1973 by Leszek Lukaszuk and Basarab Nicolescu to potentially explain differences at higher energies between proton-proton and proton-antiproton collisions due to strong interaction but until recently no convincing evidence of its existence had been presented. In QCD, the Pomeron and the Odderon correspond to the exchange of two (or even number of) and three (or odd number of) gluons as shown by Figure 2. The Odderon is not a particle in the ordinary sense since it doesn’t have a definite mass and lifetime. Instead it is a compound of gluons sufficiently bound together to be exchanged between two protons (or a proton and an antiproton) without the gluons of the Odderon interacting individually with the building blocks of the proton, the quarks and the gluons.
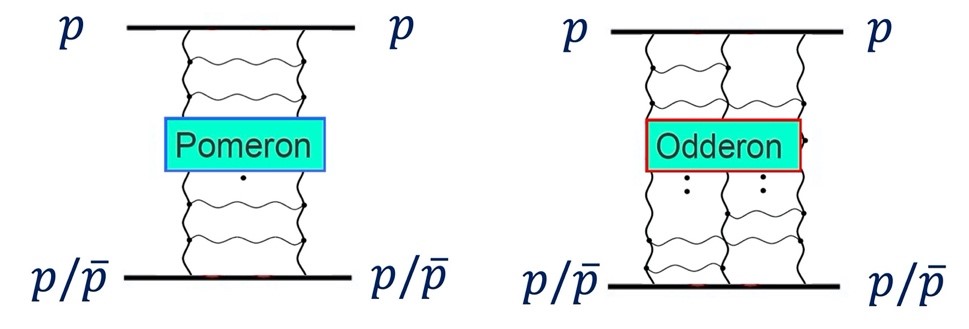
How can the Odderon be discovered?
Since the Odderon is exchanged and not produced in the proton-proton collisions, one cannot discover the Odderon by observing a “peak” or a “bump” in a mass distribution like for the Higgs boson. In addition, the Pomeron, being made up by only two gluons with matching colours, is much easier to be exchanged between particles containing gluons than the Odderon, being made up by three gluons whose colours have to match the colour-neutral condition. So two things have to be fulfilled by a process to be able to discover the Odderon: the Odderon can be exchanged and other competing exchanges, especially Pomeron exchange, have to be heavily suppressed.
The ideal process is elastic scattering and its so-called diffractive minimum. What is the diffractive minimum? The diffractive minimum is the part of the elastic scattering distribution as a function of the variable |t |, where there is a large decrease (“dip”) in the distribution followed by a significant increase. Physically it measures the size of the proton in analogy with diffraction of photons on a material surface to find out the three-dimensional periodic structure of the atoms in a crystal. What is t ? t is a variable that physicists use to describe the transfer of momentum in the collision. It is defined so that it is always negative. For convenience physicists usually use its absolute value. In elastic collisions, |t | corresponds to the square of the scattering angle, the change of direction of the proton due to the collision. So in practice, the diffractive dip is just a certain range of scattering angles, where the probability for elastic collisions to happen is much lower than in the range of scattering angles larger and smaller. Since the probability for elastic collisions is much lower, the dominant Pomeron exchange must be suppressed. Add to this the fact that the Odderon interacts oppositely with antiparticles than with particles, the way to discover of the Odderon is open. Any significant difference between elastic particle-particle scattering and elastic particle-antiparticle scattering in the diffractive dip region at the same collision energy is evidence for the Odderon. To be more precise, such a difference should be observed at very high energies, preferably above about 1 TeV, where gluonic exchanges dominate and quark-antiquark exchanges are very unlikely.
Discovery of the Odderon
In December 2020, the discovery of the Odderon was made public by the TOTEM experiment together with the D0 experiment at Fermilab’s Tevatron collider in the US. Since the LHC cannot accelerate antiprotons, the comparison was made with the existing elastic proton-antiproton measurement in the region of the diffractive dip at the highest possible collision energy, the one by the D0 experiment at 1.96 TeV. In addition, a measurement of elastic proton-proton collisions at a collision energy of 1.96 TeV in the region of the diffractive dip at the LHC is not possible due to the LHC design. That’s why the TOTEM elastic proton-proton measurements at the collision energies of 2.76, 7, 8 and 13 TeV were extrapolated to the collision energy of 1.96 TeV to make a direct comparison with the D0 measurement. In the region of the diffractive dip, the distributions disagree as can be seen in Figure 3 to such a level that the difference can only be due to Odderon exchange. This led to the discovery of the Odderon, when this new evidence was combined with a previous evidence of the Odderon from the inability of Pomeron exchange to describe the TOTEM elastic proton-proton measurements at even smaller scattering angles. The article with the discovery was published in Physical Review Letters last month (August 2021).
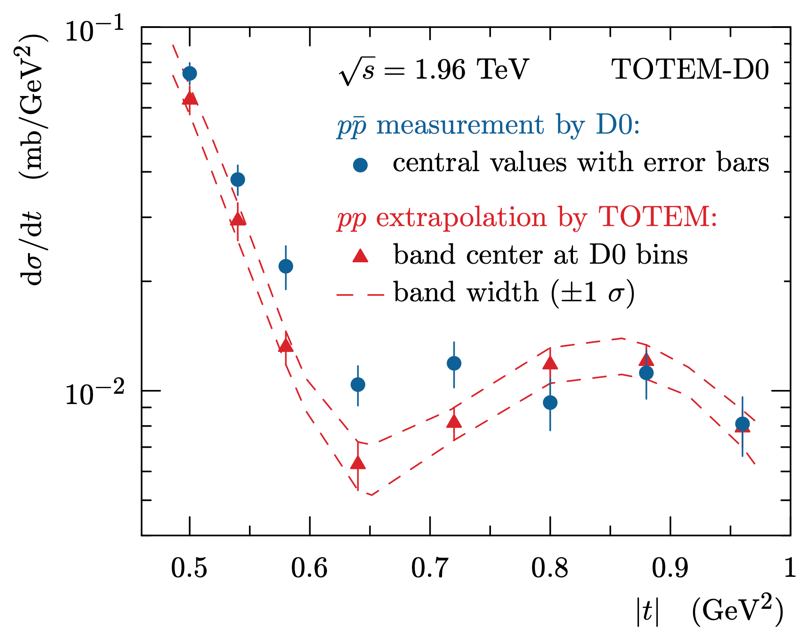
What’s the big deal?
You might think: Why should we bother? What is the big deal with the Odderon? The Particle Data Group (pdg.lbl.gov) lists hundreds of particles so a new one should not make such a difference. And it is not even a particle in the ordinary sense with a mass and a lifetime.
Well, the Odderon is different. It is not made of quarks like the large majority of the others in the Particle Data Group. In addition to the Pomeron, it is only one made only out of gluons. Its existence also suggests that real particles made only of gluons, so-called glueballs, exist, just as QCD predicts. There is still much research to be done: after establishing the existence, the Odderon should be characterised. TOTEM is pursuing measurements of the behaviour of the Odderon as function of the collision energy, complementing the existing measurements with new ones at 0.9 and 14 TeV. The LHC is also potentially a good environment to find glueballs in the gluon rich environment of central exclusive production, where the two colliding protons loose energy but stay intact and create particles in between them. TOTEM experiment is studying glueball candidates together with the CMS experiment. Stay tuned!
Kenneth Österberg
Professor in Experimental Particle Physics, University of Helsinki
Physics coordinator, TOTEM experiment, CERN