The future of physics will be seen with gravitational waves
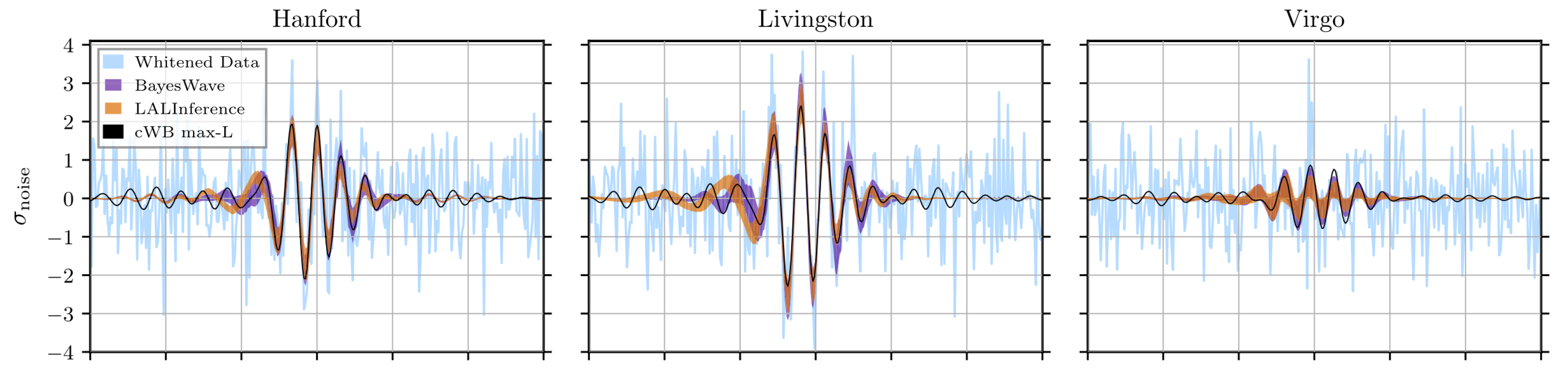
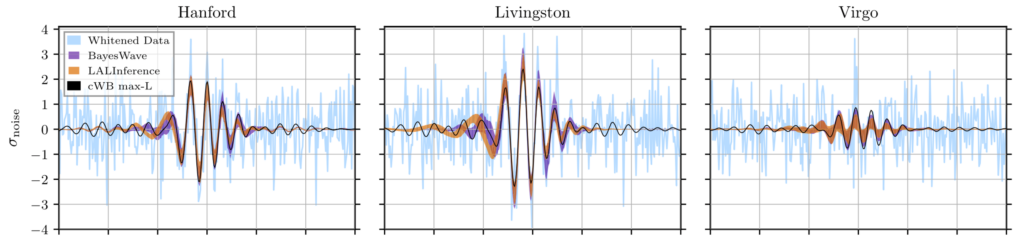
Gravitational wave amplitude as a function of time in GW190521, as observed by the three gravitational wave detectors in the LIGO/Virgo collaboration, along with the inferred signal from a merger of two black holes with masses of 85 and 66 solar masses. Each vertical line is separated by 0.05 seconds. Reproduced under Creative Commons 4.0 license from Physical Review Letters 125, 101102 (2020).
Biggest black hole merger so far
Great excitement has been generated by the recent publication by the LIGO/Virgo collaboration (LVC) of a gravitational wave burst interpreted as a merger of black holes with masses 85 and 66 times greater than that of the Sun, leaving a final black hole with mass 150 solar masses. The remnant black hole formed in this event, labelled GW190521, is the most massive detected by LVC so far. Black holes with masses in the range 65 – 130 solar masses are not expected to form from collapsing stars, so there is a puzzle about how the more massive progenitor was formed.
A puzzle – how were these black holes made?
The gap in the range of predicted masses is called the pair instability mass gap. A star with a mass of about 100 solar masses becomes so hot at its centre that the black body photons have enough energy to turn into electron-positron pairs. This reduces the pressure and the centre of the star shrinks and heats up further. If the star is not too massive, it starts pulsating and shedding material, before eventually collapsing into a black hole with mass less than about 65 solar masses. If the star is more than about 130 solar masses, the theoretical expectation is that it blows completely apart, and no black hole is formed at all.
The LVC studies various explanations in detail in a longer paper. One possibility is that at least one of the progenitors is itself the result of a merger. Another more exotic formation mechanism for black holes in the mass gap is by the collapse of a rare large density fluctuation very early in the history of the universe, into what’s called a primordial black hole. This scenario predicts black holes which do not spin, at least until they themselves merge. It’s interesting that there is evidence from the detailed shape of the gravitational wave burst that at least one of the black holes in GW190521 was spinning.
Was light from the merging black holes also seen?
A further intriguing feature of the event is an observation of a flare in an active galactic nucleus (AGN) in about the right direction and at about the right distance to be a so-called electromagnetic counterpart to the black hole merger. The flare reached peak brightness about 50 days after the merger, which could be consistent with a model in which the binary was located in a disk of hot gas surrounding a supermassive black hole. Variations in the intensity of the electromagnetic radiation from this disk is what makes an AGN “active”. This kind of coordinated observation of an astronomical object or event by several different methods is known as multi-messenger astronomy, and is an important and rapidly growing field. Perhaps the most spectacular example was the simultaneous observation by LIGO and many other instruments detecting electromagnetic radiation of a pair of merging neutron stars in 2017 (see also Aleksi Vuorinen’s blog post, June 2020) .
Nanohertz gravitational waves?
Another recent gravitational wave observation has caused a stir, this time from NANOGrav (North American Nanohertz Observatory for Gravitational Waves), which is a collaboration looking for gravitational waves by observing, over a long period, dozens of millisecond pulsars in our galaxy using radio telescopes in the US. Each one of these pulsars is a rapidly-spinning neutron star emitting radio pulses with periods of a few to tens of milliseconds. They act like a set of accurate clocks distributed around us in space. A gravitational wave passing through this net of clocks would disturb the arrival times of the ticks at the Earth in a characteristic way. In the latest NANOGrav dataset, based on 12.5 years of observations, an unexplained slow variation in the ticking has been seen, which could be gravitational waves with a period of a few years. Such long-wavelength gravitational waves would be produced by the merger of black holes of billions of solar masses, such as those known to exist at the centres of many galaxies, and recently directly observed at the centre of M87 by the Event Horizon Telescope. A more exotic possibility is oscillating loops of cosmic string, remnants of a phase transition in the very early universe.
Where next for gravitational wave detection?
Naturally, the excitement of gravitational wave astronomy is motivating investment in more detectors around the world. In Japan, KAGRA started observing this year, but as with LIGO and Virgo, had to shut down when the Covid-19 pandemic hit. A third LIGO detector is going to be built in India. In the more distant future is Einstein Telescope. But the next phase of gravitational wave astronomy will need to be out of this world. On the Earth, seismic activity limits observations to frequencies above 10 Hz or so. This means that mergers of the supermassive black holes known to exist at the centres of galaxies can’t be observed, as they radiate at a much lower frequency. To see these events, gravitational wave detection will move into space. HIP members are involved in the European Space Agency’s LISA (Laser Interferometer Space Antenna), due for launch in 2034. LISA will be a triangular arrangement of satellites using laser interferometry to measure changes in their 2.5 million km separation to an accuracy of a few picometres, enabling the instrument to detect for merging black holes of a million solar masses or more. It will also be able to listen out for gravitational waves from violent events very shortly after the Big Bang, as David Weir explained in his blog post here last year.
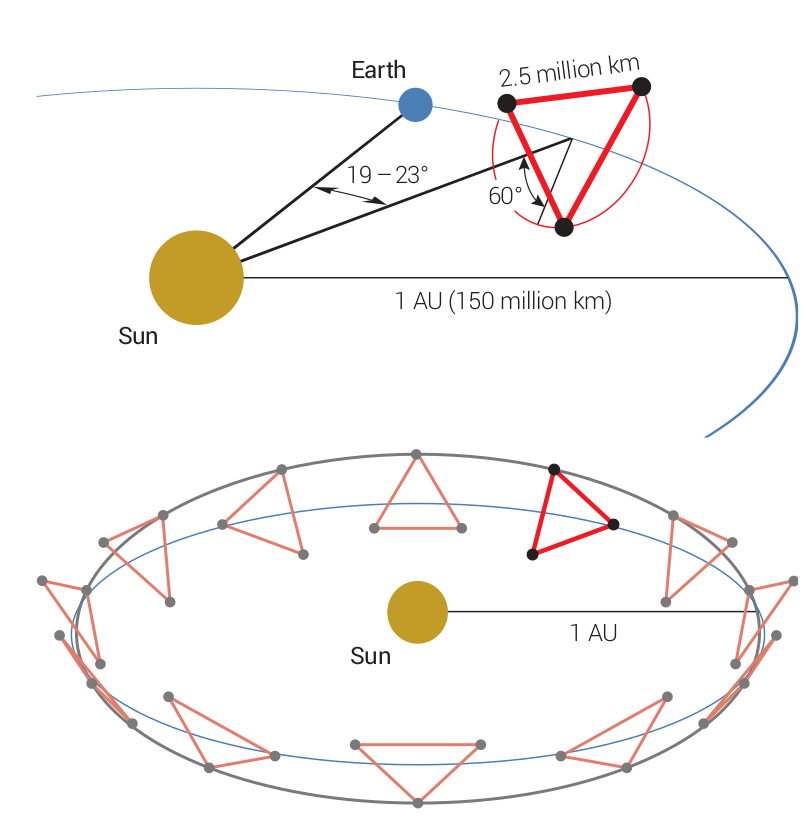
The orbit the three LISA satellites will take, trailing the Earth on its path around the Sun by about 10 million km. Reproduced from arxiv:1702.00786
LISA may also be joined by the planned Chinese space-based detectors Taiji and TianQin. Taiji is designed with a very similar specification to LISA, while TianQin would be a smaller constellation orbiting the Earth at a distance of around 100,000 km, placing its three satellites inside the Moon’s orbit. It will be less sensitive than LISA and Taiji, but it avoids some of the challenges involved in propelling satellites so far away from the Earth. Not least of these is the faintness of the radio signals coming from LISA, which will mean that the data transfer rate will be no better than that of a late 1980s modem.
Seeing the future with gravitational collaboration
Extraordinary discoveries have already been made with gravitational waves, and there will surely be many more. One of the most exciting things about the field is that it brings together scientists working in quite different fields, as the observation of the binary neutron star merger has already shown. Theoretical physicists, like me, trying to use the gravitational echoes of a bubbling universe at around 10 picoseconds after the Big Bang to probe the fundamental nature of matter have a shared interest with astronomers seeking to understand the life-cycle of the huge black holes at the centres of galaxies. Both goals critically depend on the analysis of gravitational waves of frequencies around a millihertz, where LISA will be most sensitive. We will need to work together to disentangle the signals, to learn about the parts of the universe most distant in space and time. This is also perhaps where new laws of physics are hiding.
Mark Hindmarsh
I thank colleagues in Helsinki and elsewhere in Finland for helpful discussions about these exciting results.