Central exclusive physics at LHC: a look forward

We are surrounded by them, composed of them, constantly interacting with them. However with their complex structure and behaviour, protons are still covering a large fraction of the uncharted territory of modern particle physics.
Few classes of instruments allow to probe its constituents with high precision. Among the man-made flavour, the Large Hadron Collider at CERN became widely known to be smashing a few billions of them every fraction of a second (precisely, in normal proton-proton operation mode, bunches of about 1.15×1011 protons are collided every 25 nanosecond) in large detectors under the Rhône plain stretching between Switzerland and France. A handful of physicists are particularly interested in studying the tiny fraction of all possible reactions where protons are surviving this violent encounter. At HIP, this interest is materialised with of a team of half a dozen researchers partaking on both the theoretical and experimental fronts.
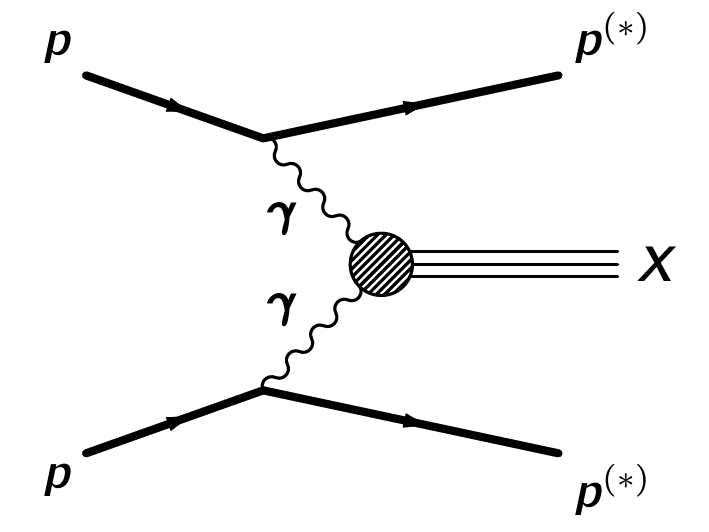
For the theoretical part, a lasting international collaboration allows the development and maintenance of a tool to estimate numerically the contribution of multiple photon-induced (and more generally central exclusive, and diffractive) processes in proton-proton and heavy ions collisions. Through the factorisation from fluxes of photon emitted from the proton of the central matrix element describing the two-photon process under study, increasingly complex final states can be modelled. These comprise fermion, vector boson, or supersymmetric particles pair production. Its originality with respect to “traditional” equivalent photon approximation principles commonly used to describe this class of processes lies in its transverse-momentum dependent modelling of both the intermediate particle fluxes and matrix elements. This framework also allows to account for multiple kinematic features arising from the non-empty probability of protons to dissociate even in cases of elastic photon emission. It hence provides an interesting toolbox for both the phenomenological and experimental communities to study the effect of proton survival factors at unprecedented energy scales.
On the experimental front, HIP is involved in the development, operation, maintenance, and data analysis of the precision proton spectrometer (PPS), a joint collaboration between the CMS and TOTEM experiments at CERN. Located at about 220 metres on each side of the CMS detector, PPS hosts an array of various technologies of near-beam detectors designed to tag and localise scattered protons moving in close vicinity from the LHC primary beam. A good fraction of central exclusive reactions, this particular class of interactions where only colour-singlet objects (such as photons) are exchanged, involves the “survival” of both protons interacting. Experimentally, this provides a striking signature of a central system with exclusive-like kinematic features, and two leading protons to be observed downstream of the collision point. In PPS, both tracking and timing detectors are embedded in so-called Roman pots stations for proton detection.

The tracking component consists of silicon sensors (initially edgeless strips as used in TOTEM, then arrays of 3D pixels as developed for the CMS central tracker, with a finer spatial resolution and radiation hardness), allowing the reconstruction of proton transverse positions. Combined with the precise knowledge of optical properties of the LHC beamline elements located between the spectrometer and the proton-proton interaction point, it provides the proton longitudinal momentum loss ξ and the single-sided virtuality t = -q² (well know since the era of deep inelastic scattering experiments at e.g. SLAC or HERA).
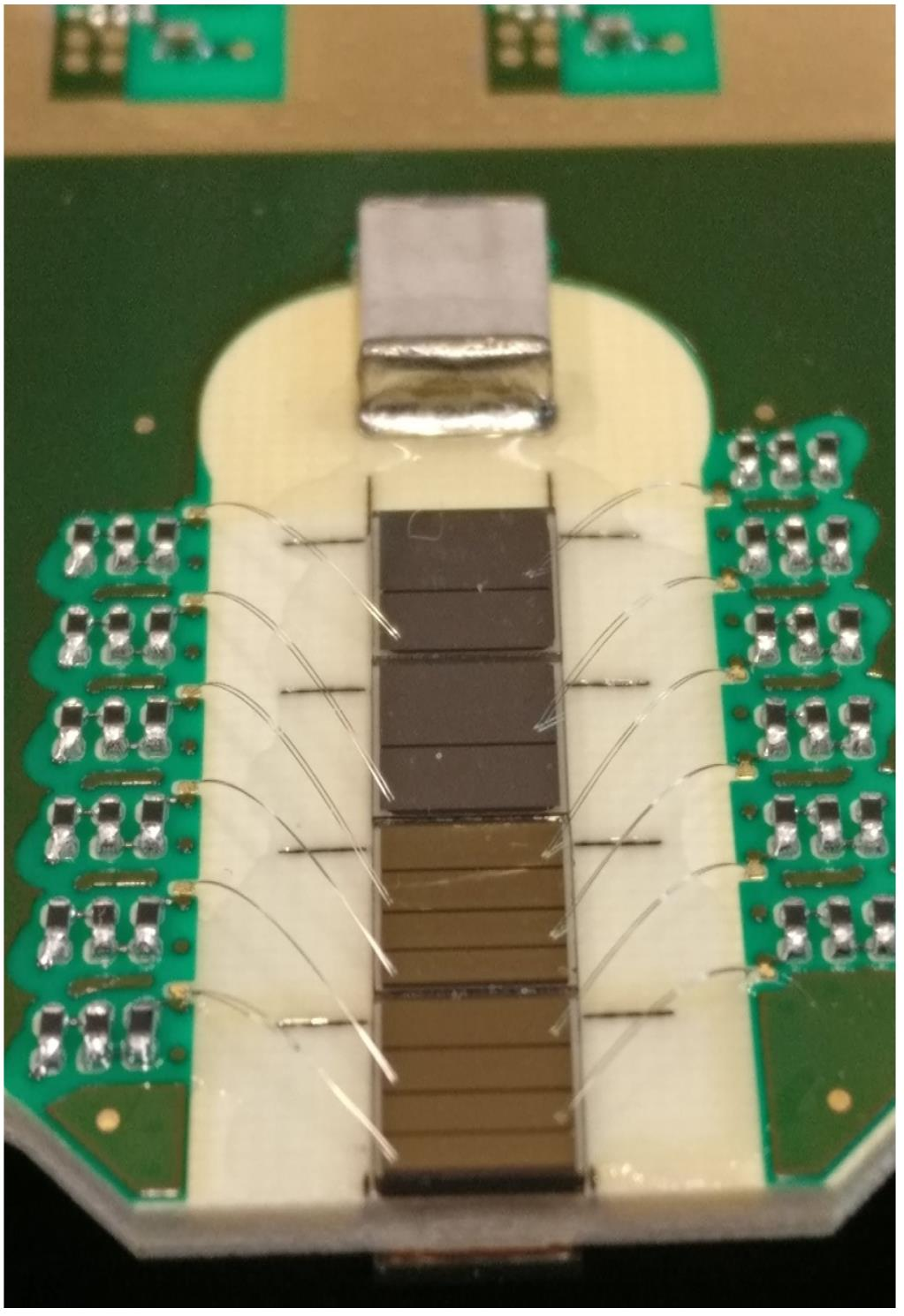
The second PPS component acts as a time of flight detector, where a measurement of the arrival time difference between the two protons (in the case of fully exclusive production) determines the longitudinal coordinate of the collision point. With a precision of about 2 mm per 10 picosecond of time precision, a large suppression of secondary interactions occurring at each crossing of the proton bunches (often referred to as pileup) can be expected from well performing sensors. At HIP, half a dozen researchers are involved in the R&D, testing, characterisation (thanks to a campaign of test beams operated at DESY-II during the second long shutdown of CERN accelerators chain), and commissioning of existing and new single-crystal chemical vapour deposition (scCVD) diamond sensors. Already reaching a timing precision of about 100 ps per proton track as measured in 2018, thanks to an innovative calibration procedure developed for the full readout chain [1], a second station will be installed on both arms at the dawn of LHC’s Run 3 (2021-2023). HIP is in charge of the procurement, quality testing, and integration of the new sensors in the PPS environment, involving a big fraction of the HIP CMS upgrade project members.
In terms of research output, using only a small fraction of the 110 fb-1 collected in 2016-2018 at 13 TeV, the CMS-TOTEM PPS has already demonstrated its ability to operate continuously a near-beam proton spectrometer at nominal LHC luminosity rates through the observation of high-mass lepton pairs correlated with forward proton tracks [2]. This result, still not exploiting the good performances of timing detectors, will soon be joined with additional, more complex final states thanks to the outstanding performance of PPS along LHC’s Run 2.
Laurent Forthomme
Postdoctoral researcher.
Department of Physics and HIP, University of Helsinki
[1] CERN-CMS-DP-2019-034. CERN, Geneva, 2019.
[2] JHEP 07 (2018) 153; e-Print: arXiv:1803.04496 [hep-ex]